Quantum sensors show how physics-based tech is yielding federal advances
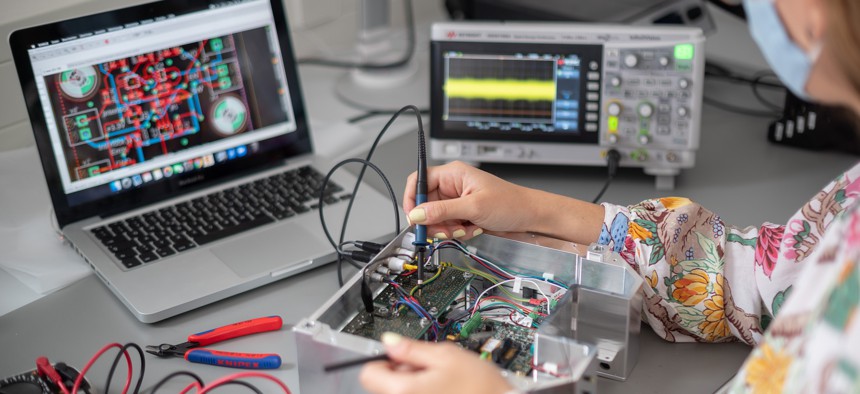
A laboratory worker at the German technology firm Q.ANT shows a voltage measurement on a quantum sensor. Quantum sensing has been around for decades, but scientific and engineering advances are generating new use cases for the technology. Sebastian Gollnow/picture alliance via Getty Images
True quantum computers are a long way off. But their lesser known cousins — quantum sensors — are already paying dividends in the federal scientific communities.
Among the emerging technologies federal agencies are beginning to grapple with, quantum computing has captivated imaginations. Much like artificial intelligence and biotechnologies, quantum computing — the new, powerful genre of computer systems that operate on the principles of quantum physics — presents both public and private sectors with hope and hazard.
This attention is broadly due to the theoretical risk a fault tolerant quantum computer –– which does not exist yet –– could pose to current data assets housed on more vulnerable classical computers. In turn, federal agencies have responded with proactive efforts to safeguard data and digital networks on a cryptographic level.
For all of the hype surrounding quantum computing, there has been another quantum information system long in existence that receives a fraction of the attention: quantum sensing. Like any other quantum technology, quantum sensors marry the principles of quantum mechanics into existing sensing technologies. Their advantage focuses on delivering precise and reliable measurements into the world around us by tracing changes in the invisible atomic world.
“Sensors have been around for a huge amount of time,” Yaakov Weinstein, the director of quantum technologies at the MITRE Corporation, told Nextgov/FCW. “For a quantum computer, if you're dealing with atoms, you need to address single, individual atoms. With a quantum sensor, you don't actually need to.”
Adam Black, the section head for quantum optics at the U.S. Naval Research Laboratory, told Nextgov/FCW that the quantum-powered sensors take established sensing technologies and utilize atoms, the fundamental building blocks of all matter, to accurately measure spatial factors like time, longitude and latitude, and distance.
“You don't necessarily require quantum mechanics to make very good sensors,” Black said. “But it was recognized in the 20th century that quantum mechanical systems — particularly atoms, but other types of systems as well — provide an opportunity to make more accurate sensors than were achievable through classical technologies.”
The initial need for sensing technologies grew from a desire to measure one’s surroundings beyond what was available to humans’ normal senses. Weinstein said that embedding atoms into sensing technologies is an optimal solution given the natural sensitivity of such particles to changes in environmental energy levels. Put simply: quantum sensors rely on atoms’ inherent tendency to change behavior based on minute differences in their environment to measure things that other systems don’t have the sensitivity to detect.
This has birthed applications currently used in subterranean navigation, radar target tracking and identifying, onshore drilling efforts, and more, Elliott Mason, a patent agent at Young Basile, told Nextgov/FCW.
“Atoms can be affected by all sorts of different forces,” Weinstein said.
One of the most well-known examples of quantum-enhanced sensors in action is the atomic clock. Instead of relying on the classical architecture of clocks that risked manufacturing and programming inconsistencies leading to different outputs of time, atomic clocks measure the frequency levels between the electrons pulsating around atoms like waves. Reading these particle fluctuations provides an objective interpretation of changes in the clock’s surroundings.
Black added that the uniformity of atomic structures, the fundamental laws that govern reality, serve as the reference point for clocks that prevents inaccuracy in their frequency readings. He explained that by detecting the minute shifts in internal subatomic energy level changes, atomic clocks tell time in a more precise manner than their quartz-based and mechanical counterparts.
The crux: atomic clocks read the environmental changes stemming from the invisible quantum energy changes, taking advantage of benchmarking time against natural environmental frequency readings.
“We no longer have to worry about how accurately we, with our two hands, put together our fundamental frequency reference [to measure time],” he said. “We now can let nature take care of that really hard part for us.”
This operation itself relies on other supporting technology. Depending on the specific sensor system, that could include vacuum chamber, lasers, microwave sources and more. Fundamentally, however, the sensor is able to offer measurements based on “unchanging natural phenomenon.”
“That's what quantum mechanics provides us with,” Black said.
GPS is another arena that stands to benefit from applying principles of quantum physics. Black described an ongoing effort within the NRL to help the Navy in situations where connectivity is not available.
“There's a continuing need for ever-lower error in that navigation that can be attained without GPS,” Black said. “And so quantum technologies provide one avenue for that.”
In response, the NRL developed an architecture to measure a given vessel’s accelerations and rotations using a continuous beam of laser-cooled atoms. While at such a low temperature — about 10 millionths of a kelvin above absolute zero — Black and other researchers can manipulate the atoms through a vacuum cell via a process called atom interferometry.
“This technique uses the fact that, quantum mechanically, atoms are both particles and waves, and they can interfere within themselves and produce [a] certain interference pattern that we can measure,” Black said. “And from the interference pattern very accurately measure how the sensor that the atoms reside in is accelerating and rotating.”
That measurement can then tell operators exactly where they are in relation to their surroundings.
Laboratory demonstrations have been “very successful” so far, according to Black. Now researchers are trying to prove its efficacy in a shipboard demonstration within the next two years.
In the future, these systems could also prove useful below the surface.
“It's accurate that extending mission duration without a need for GPS is an important goal of these quantum inertial sensing technologies…for submarines,” Black said.
At MITRE, Weinstein told Nextgov/FCW that current sensing research works on leveraging the unique electron structure of Rydberg atoms — which are in a high energy state — to create an electric field sensor, another emerging system that could form more efficient antennas and a more resilient telecommunications infrastructure.
“It turns out to be very useful for sensing electric fields, like antennas, but not very good at transmitting electrical signals,” Weinstein said.
With such advantageous use cases, the natural next step for quantum-enabled sensor technologies is commercialization. Black explained that quantum magnetometers are one such commercially available product, along with smaller atomic clocks, which have been on the market since approximately the late 1950s, thanks to private and public sector collaboration. Atomic gravimeters, which measure the strength of a given gravitational field and can be useful for subterranean exploration, are more limited commercially but are still available.
Black noted, however, that quantum information technologies are still in their relative infancy. He highlighted other emerging systems, namely integrated photonics and properties of quantum entanglement, as two potential aids to further improve these sensing technologies.
“There's a great deal of engineering that's involved in taking these things from the lab, to fieldable prototypes and then commercial products,” he said. “So there's a kind of an ongoing progression of commercialization as the different basic science developed and [was] demonstrated in the laboratory and as the different supporting components reach technological maturity.”
On the public research and funding side, agencies are paying attention to the potential trajectories of quantum sensing systems. In 2023, the National Science Foundation announced a new $29 million investment in quantum sensing research. More recently in April, a bicameral duo of Republican lawmakers proposed a bill to organize a more robust quantum information research infrastructure within the Department of Defense with the hope of furthering innovation in the field.
Much of the federal research capabilities are still hinging on the renewal of the National Quantum Initiative Act, a 2018 law that prioritizes funding quantum information sciences research and development within the federal government. It officially authorized $1.275 billion in funding over five years.
As its reauthorization is still pending approval by House lawmakers, industry advocates like the U.S. Chamber of Commerce are voicing support for its passage, citing national security concerns.
“The nations that lead in quantum development and commercialization will reap a global strategic advantage. Other countries – especially China – are making significant investments in this area and are challenging U.S. leadership in this realm,” a cohort of advocacy groups wrote in a statement released on Monday. “We strongly support the National Quantum Initiative Reauthorization Act and respectfully ask that it be enacted this year.”
Editor's note: This article has been updated to reflect the temperature measurement of the NRL architecture for laser-cooled atoms.